This image of natural cuprite (Cu2O) was acquired at 74x magnification, which is near the 50x lower limit of magnification for our instrument. It shows the general acicular to fibrous nature of the material seen at low magnification.
Imaging
Available Signals
Five signal types are available for imaging on our electron microprobe: secondary electrons, backscattered electrons, x-ray intensity, absorbed current, and cathodoluminescence. Descriptions of the available signals are given below, along with examples of images and their applications for most signal types.
Secondary electrons are low energy electrons emitted from very near the sample surface. This signal provides an image of the sample topography, and hence, external morphology. This can be applied not only to simple characterization of a sample material but also to a variety of other applications including component failure analysis or the determination of chemical stability of materials indicated by growth or dissolution features.
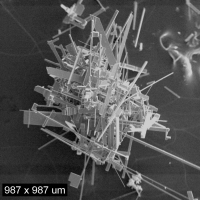
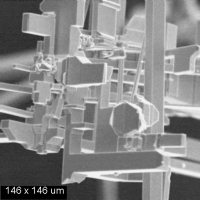
This image of the same cuprite was acquired at 500x magnification, which is near the limit of resolution by typical far-field optical microscopy. It shows the reticulated habit of grains that appear prismatic at lower magnification.
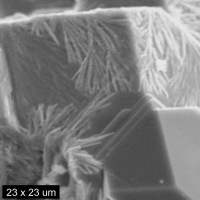
This image of the same cuprite was acquired at 3000x magnification, which exceeds the resolution of typical optical microscopy. It shows that grains appearing smooth at lower magnification are coated by fine, dendritic to radial skeletal growth.
Backscattered electrons have higher energies than secondaries, and are produced when electrons from the primary beam are "bounced" back out of the sample by elastic collisions with atoms. The number of electrons a given atom will backscatter is proportional to its mean atomic number. Materials composed of larger, heavier atoms will backscatter more electrons, producing brighter gray tones in the images than less dense materials (differences in average atomic mass of 0.1 amu can be resolved). Backscattered electrons thus produce an image that is related to material composition, providing both spatial and chemical information. This signal is especially useful for characterizing fine-grained multicomponent materials (first two images, below) and for documenting chemical heterogeneity in single coherent phases (third image, below).
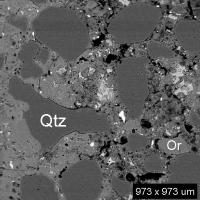
This is a low magnification BSE image of mortar from the brickwork of the Sarkeys energy center. It shows the general structure of the mortar including fine aggregate comprised of quartz (Qtz) and orthoclase (Or) sand grains plus minor hematite (Fe2O3: white, near center of image), set in a matrix of Ca- and Al-silicates. Note the heterogeneity within the matrix, especially with respect to the porosity gradient from the more massive high-Ca cement at the left to the more variable and hydrous Si-rich cements at the right.
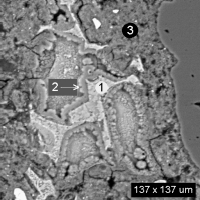
This image is a detail from near the of the center of the previous image, and shows heterogeneity within the Si-rich cement defined by sequential formation of (1) early ferruginous di-calcium aluminate, followed by (2) di-calcium silicate, and finally very hydrous low-calcium silicates (3).
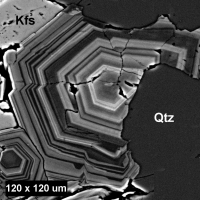
This image is of two banded tourmaline crystals with quartz (Qtz) and orthoclase (Kfs) from a granitic rock. Banding in the tourmaline, nominally Na(Fe,Mg)3Al6(BO3)2Si8O18(OH,F)2, is due exclusively to variations in the Fe:Mg ratio (the Fe-rich zones are lighter), as the molecular fractions of other components in these crystals are essentially constant.
Elemental Distributions. X-ray intensity images document the distribution of selected elements in a material. Therefore, they can show the chemical basis for intensity variations observed by BSEI. X-ray images can be acquired with either or both the WDS and EDXA detectors. Images can be calibrated to produce quantitative elemental distribution maps, either by automated process during acquisition or manually after acquisition if the concentrations for two points of different intensity are known. Image output can be in color or black/white, and color schemes for the images can be user-defined. In the following example of a metallurgical slag, brighter colors indicate higher intensities (red = max, black = min). The BSE image (upper left) shows the complexity of the slag in which the four most abundant phases, in order of decreasing average atomic mass are: (1) metallic Fe (white in BSEI: note the high intensity in the Fe Ka image, and no intensity in the O Ka image); (2) skeletal, apparently cubic (Cr, Mn)-oxide (light gray in BSEI: note the high intensities in the Cr Ka image, and low intensity (blue) in the O Ka image); (3) interstitial Cr-aluminosilicate that is probably quenched to glass (dark gray in BSEI: note low intensity in Cr Ka image, but moderate intensity in Si Ka and high intensity in Al Ka images) and (4) silica (SiO2) that is likely quartz (black in BSEI, high red intensities in the Si Ka and O Ka images.
Resolution of phases with similar electron backscattering cross-sections. In some cases, different phases have very similar average atomic masses, and may be difficult or impossible to distinguish by BSEI. In such cases, even subtle differences in composition between the phases may permit their discrimination by x-ray imaging.
The example below shows three images of an experimental product from a high tempeature study of crystal growth from granitic melt. The left BSEI shows patchy intergrowth of potassic alkali feldspar ((K,Na)AlSi3O8): white) with albite feldspar (NaAlSi3O8: medium gray) and quartz (SiO2: medium gray) in melt that was quenched to glass (darker gray, lower right corner of image). Because albite and quartz have very similar electron backscattering cross sections, they are often difficult to distinguish by BSEI. This distinction is easily made, however, by the use of x-ray imaging. The center image is of Na distribution: the red areas are richest in Na and, hence, are albite. The right image shows Si distribution; the red areas are quartz which is the phase having the highest Si content in this system.
![]() | ![]() | ![]() |
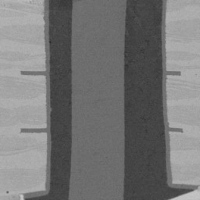
Absorbed current images show differences in electrical conductivity within a sample. As such, the signal can be used to examine variations in composition or structure, especially in conductive materials like metal alloys and electrical components like this component socket (solder fillet) in a printed circuit board.
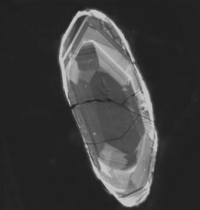
Cathodoluminescence (CL) is the emission of energy in the form of light in the UV to near-IR (including the visible wavelengths) produced by many materials when bombarded by high energy sources, which is an electron beam in our instrument. For many or most such substances, the color and intensity of the cathodoluminescent signal are very sensitive to trace element chemistry and/or defects in the mineral lattice. This makes the CL signal very useful for distinguishing zoning in crystals that may not be resolved by optical microscopy or BSEI. This can be applied to growth history and kinetics for many minerals or synthetic compounds, especially as a guide to selecting points for quantitative analysis of minor to trace level components by WDS or other laser- or ion-beam methods.
The following CL image of a U-rich zircon crystal in epoxy was acquired in about 1.5 minutes using a 5 nA beam current. Note that lattice damage caused by the decay of radioactive uranium diminishes cathodoluminescence intensity in zircon, causing weak luminescence in this grain (many zones show CL intensity comparable to, or weaker than, the surrounding epoxy).
Imaging Methods
Images, especially those utilizing electron or absorbed current signals, are typically viewed in live-time on a 17" display monitor much like those of standard SVGA computer displays; two video channels are available. The rate of beam scanning can be varied from TV rate to 12 seconds per frame. Live-time images are typically captured directly to device independent bitmap (BMP) digital file that provide no data loss due to image compression, but can be converted to a variety of other formats providing great flexibility, exportability, and opportunities for image enhancement and analysis.
Image Enhancement, Analysis, and Output
A variety of software packages are available for simple viewing and enhancement of digital images. Among these Media Cybernetics Image Pro Plus® runs as an off-line solutions, supporting intensity and spatial filtering, arithmetic operators, and text annotation. Image Pro Plus® has significantly greater capabilities for text annotation and image manipulation, and thus most enhancement and editing is performed with this package using standard (TIFF, JPEG, GIF, BMP) file formats.
Quantitative image analysis is performed using the Media Cybernetics Image Pro Plus® package. In addition to image enhancement capabilities, this package supports a wide array of features for deriving quantitative information from images. Among those features are included: manual and semi-automated object measurement (size, intensity, shape, orientation), classification, and counting, with result analysis and statistics exportable to spreadsheets; spatial and intensity calibrations with corrections for non-linearity; spatial, logical, arithmetic, and background correction operations; false coloration of gray-tone images, true 24-bit color processing of RGB images, and color-based image segmentation; and capabilities for simultaneously working with multiple images. When used in concert with logical (Boolean) and arithmetic operators, the latter feature can be extremely useful for producing phase distribution maps in complex multi-component systems from combinations of x-ray intensity and backscattered electron images.
Image output can be either to hardcopy or digital file (a large variety of file formats are supported including TIFF, JPEG, GIF, and many others).